On this page
Introduction
PM2.5 has become a significant public concern in recent years. In 2012, the Chinese government revised the "Ambient Air Quality Standards," adding PM2.5 monitoring and control requirements and implementing these standards in major cities nationwide. Currently, the main methods for monitoring particulate matter include the gravimetric method, micro-oscillatory balance, β-ray absorption, and light scattering. The gravimetric method is widely accepted as a standard due to its simplicity and minimal influencing factors, and it serves as a reference for validating other monitoring methods. Traditional PM2.5 monitoring methods are accurate but often involve bulky, noisy, and cumbersome instruments, limiting their practicality for indoor air monitoring. With advancements in wireless sensor technology, PM2.5 sensors are developing rapidly. They offer advantages such as quick response, high sensitivity, real-time performance, compact size, and low cost, making them valuable for indoor air quality monitoring. However, few studies have focused on sensor monitoring performance and its influencing factors, leaving uncertainty about comparability across different sensor models. Therefore, studying the relationship between sensor measurements and standard gravimetric instrument results is essential. This study uses gravimetric instruments and sensors from various brands to sample and monitor indoor air PM2.5. By comparing and analyzing the results, we evaluate the monitoring performance of PM2.5 sensors and offer insights for advancing PM2.5 monitoring technology based on wireless sensor systems.
1. Experimental Method
1.1 Experimental Instruments
The instruments used for the gravimetric method included an intelligent particulate sampler (Qingdao Laoying 2050D, with a sampling flow rate of 100 L/min), a 90mm glass fiber filter membrane, and an analytical balance (Sartorius CPA26P, Germany, with a sensitivity of 0.01 mg/0.002 mg). For PM2.5 sensors, five different brands based on the light scattering method were selected and are referred to below as A, B, C, D, and E.
1.2 Experimental Method
Indoor PM2.5 concentrations were monitored in a laboratory setting from September to November 2015. Doors and windows were kept closed, and there was no noticeable indoor pollution source. Five PM2.5 sensors and a gravimetric sampler were placed at the same height (1.5 meters above the ground) and sampled in parallel. The center distances between each instrument's air inlet ranged from 30 to 50 cm.
Based on PM2.5 concentration ranges, the experimental design included four working conditions: (1) <35 μg/m³, (2) 35–75 μg/m³, (3) 75–150 μg/m³, and (4) >150 μg/m³. Each working condition was tested for 1–2 days, with data collected hourly. For each condition, at least six sets of valid data were obtained for analysis. PM2.5 weight was measured following the national standard "Determination of PM10 and PM2.5 in Ambient Air - Gravimetric Method" (HJ618-2011). During sampling, ambient temperature and relative humidity were recorded, with temperature ranges of 19–30°C and relative humidity ranges of 40–90%.
Statistical methods were used to analyze the correlation between the sensor and gravimetric instrument measurements, using SPSS 19.0 software. The accuracy, precision, and total uncertainty of the sensor method were calculated to assess the PM2.5 sensor’s monitoring performance. Total uncertainty was evaluated according to the health industry standard, "Determination of Inhalable Particulate Matter (PM10) in Public Places - Light Scattering Method" (WS/T 206-2001).
Based on PM2.5 concentration ranges, the experimental design included four working conditions: (1) <35 μg/m³, (2) 35–75 μg/m³, (3) 75–150 μg/m³, and (4) >150 μg/m³. Each working condition was tested for 1–2 days, with data collected hourly. For each condition, at least six sets of valid data were obtained for analysis. PM2.5 weight was measured following the national standard "Determination of PM10 and PM2.5 in Ambient Air - Gravimetric Method" (HJ618-2011). During sampling, ambient temperature and relative humidity were recorded, with temperature ranges of 19–30°C and relative humidity ranges of 40–90%.
Statistical methods were used to analyze the correlation between the sensor and gravimetric instrument measurements, using SPSS 19.0 software. The accuracy, precision, and total uncertainty of the sensor method were calculated to assess the PM2.5 sensor’s monitoring performance. Total uncertainty was evaluated according to the health industry standard, "Determination of Inhalable Particulate Matter (PM10) in Public Places - Light Scattering Method" (WS/T 206-2001).
2. Results and Discussion
2.1 PM2.5 Concentration Monitoring Results
The PM2.5 concentration monitoring results from the gravimetric sampler and five PM2.5 sensors under four working conditions are shown in Table 1. The gravimetric method recorded PM2.5 concentrations ranging from 11.04 to 388.51 μg/m³. Sensor readings ranged as follows: A (8.62–201.69 μg/m³), B (8.64–196.5 μg/m³), C (14.61–211.26 μg/m³), D (7.94–147.02 μg/m³), and E (9.63–271.20 μg/m³). Pearson correlation analysis revealed significant positive correlations between the gravimetric method and each of the five sensors, with correlation coefficients as follows: Sensor A (r=0.914, p=0.000), Sensor B (r=0.843, p=0.000), Sensor C (r=0.749, p=0.000), Sensor D (r=0.681, p=0.000), and Sensor E (r=0.857, p=0.000). Sensor A showed the highest correlation coefficient, while Sensor D had the lowest.
Table 1 PM2.5 Concentration Monitoring Results
Table 1 PM2.5 Concentration Monitoring Results
Working Condition | Method | Number of Samples (N) | Mean (μg/m³) | Standard Deviation (SD, μg/m³) | Minimum (μg/m³) | Maximum (μg/m³) |
Working Condition 1 | Weight method | 12 | 21.78 | 5.53 | 11.04 | 32.61 |
A | 12 | 21.85 | 5.23 | 8.62 | 27.54 | |
B | 12 | 19.00 | 5.21 | 8.64 | 25.47 | |
C | 12 | 35.98 | 9.05 | 14.61 | 46.07 | |
D | 12 | 26.87 | 8.18 | 7.94 | 37.28 | |
E | 12 | 21.77 | 8.14 | 9.63 | 37.79 | |
Working Condition 2 | Weight method | 14 | 50.00 | 14.36 | 34.77 | 73.47 |
A | 14 | 41.31 | 16.94 | 23.31 | 83.00 | |
B | 14 | 44.18 | 19.21 | 19.73 | 93.26 | |
C | 14 | 68.16 | 26.48 | 26.48 | 139.23 | |
D | 14 | 41.31 | 12.23 | 12.23 | 76.70 | |
E | 14 | 54.43 | 33.65 | 16.79 | 140.20 | |
Working Condition 3 | Weight method | 14 | 99.47 | 15.96 | 76.99 | 128.19 |
A | 14 | 60.03 | 20.09 | 39.77 | 109.08 | |
B | 14 | 83.30 | 25.36 | 43.01 | 126.09 | |
C | 14 | 122.24 | 42.39 | 90.97 | 178.75 | |
D | 14 | 73.29 | 27.91 | 38.22 | 111.96 | |
E | 12 | 114.11 | 38.24 | 56.70 | 166.54 | |
Working Condition 4 | Weight method | 8 | 223.82 | 74.50 | 161.46 | 388.51 |
A | 8 | 117.53 | 40.30 | 77.38 | 201.69 | |
B | 8 | 120.99 | 47.78 | 63.66 | 196.50 | |
C | 8 | 149.21 | 52.95 | 87.30 | 221.26 | |
D | 8 | 86.98 | 48.29 | 43.75 | 147.02 | |
E | 8 | 181.37 | 60.69 | 107.43 | 271.20 |
2.3 Precision Analysis
Relative standard deviation (RSD) is used to assess the measurement error in repeated tests of each sensor and to evaluate sensor precision. PM2.5 concentration data was analyzed, and the results are presented in Figure 2. Under Working Condition 1, with PM2.5 concentrations below 35 μg/m³, the RSDs for sensors A, B, C, D, and E are 24%, 27%, 25%, 30%, and 37%, respectively. Under Working Condition 2, with PM2.5 concentrations between 35 and 75 μg/m³, the RSDs are 41%, 43%, 39%, 30%, and 62%, respectively. Under Working Condition 3, with PM2.5 concentrations between 75 and 150 μg/m³, the RSDs are 33%, 30%, 35%, 38%, and 34%, respectively. Under Working Condition 4, with PM2.5 concentrations above 150 μg/m³, the RSDs for sensors A, B, C, D, and E are 34%, 39%, 35%, 56%, and 33%, respectively.
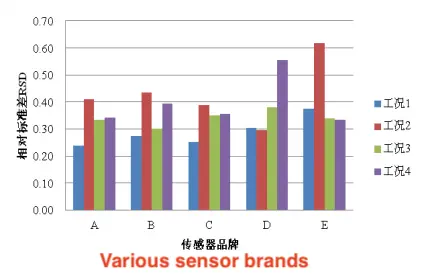
Figure 2 PM2.5 measured by sensors under different working conditions
The relative standard deviation (RSD) for the five PM2.5 sensor brands exceeds 20% under all four working conditions. The reproducibility error in sensor measurements is high, indicating low precision. For PM2.5 concentrations above 35 μg/m³, the RSD for sensors A, B, C, D, and E is 30% or higher. Because PM2.5 concentration values vary within a range for each working condition, this fluctuation may contribute to the sensors' low precision.
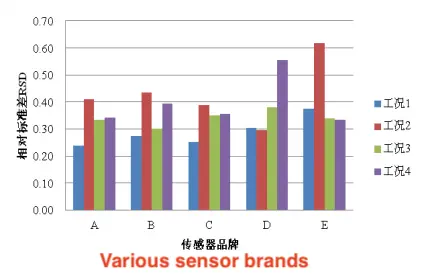
Figure 2 PM2.5 measured by sensors under different working conditions
The relative standard deviation (RSD) for the five PM2.5 sensor brands exceeds 20% under all four working conditions. The reproducibility error in sensor measurements is high, indicating low precision. For PM2.5 concentrations above 35 μg/m³, the RSD for sensors A, B, C, D, and E is 30% or higher. Because PM2.5 concentration values vary within a range for each working condition, this fluctuation may contribute to the sensors' low precision.
3. Conclusion
PM2.5 monitoring has been included in national environmental standards, and indoor PM2.5 pollution is receiving increasing attention. The development of convenient and rapid monitoring technology is an inevitable trend. Since measurement results from various instruments and monitoring methods may differ, comparative testing of these methods is particularly important. This study selected five different brands of PM2.5 sensors to monitor indoor PM2.5 concentration simultaneously, comparing them with a gravimetric instrument and evaluating their monitoring performance. The results show that the five sensors generally align well with the gravimetric method; sensor E demonstrates good accuracy, the average relative deviations of sensors B and D are within 20%, while those of A and C exceed 20%. The precision and total uncertainty of all five sensors are low, with total uncertainty significantly exceeding standard requirements. Although PM2.5 sensors are compact and convenient, many technical issues remain to be addressed in indoor environmental monitoring applications. The results of this study may have been affected by relative humidity. Studies have reported that particle concentration measurements based on the light scattering method are significantly influenced by relative humidity.
Previous: Achieving Healthy Indoor Air: Seven Essentials for Optimal Air Quality
Next: Sensors in Agriculture: Revolutionizing Modern Farming